COVERAGE
Sensuron enables real-time structural health monitoring onboard NASA’s X-56 UAV
This article appears in Aerospace & Defense Technology Magazine.
In contrast to the stiff, rigid wings found on most commercial aircraft, flexible wing technology is considered essential to next generation, fuel efficient aircraft. However, flexible wings are susceptible to “flutter,” or highly destructive aeroelastic instability.
The Lockheed Martin X-56A multi-utility technology testbed (MUTT) unmanned aerial vehicle (UAV) – a modular high-altitude, long-endurance (HALE) technology demonstrator – was specifically designed with long, flexible, very high-aspect ratio wings in order to investigate and test active flutter suppression technology.
To better understand and mitigate flutter, engineers at NASA’s Armstrong Flight Research Center (AFRC) equipped the X-56A UAV, or “drone,” with fiber optic sensing (FOS) technology.
Watch the ‘X-56A: Breaking the Flutter Barrier’ video below to learn about flutter.
For the last decade, AFRC has utilized FOS technology to perform distributed strain sensing and real time structural health monitoring during flight. Compared to traditional sensors, FOS technology provides an unprecedented level of insight into the behavior of a structure: a single hair-like optical fiber spanning up to 40 feet can act similarly to 2,000 or more strain gauges without the cumbersome and weight prohibitive instrumentation wire.
In addition to strain, FOS can be used to measure temperature, deflection, stress, load, stiffness, and various other critical engineering parameters.
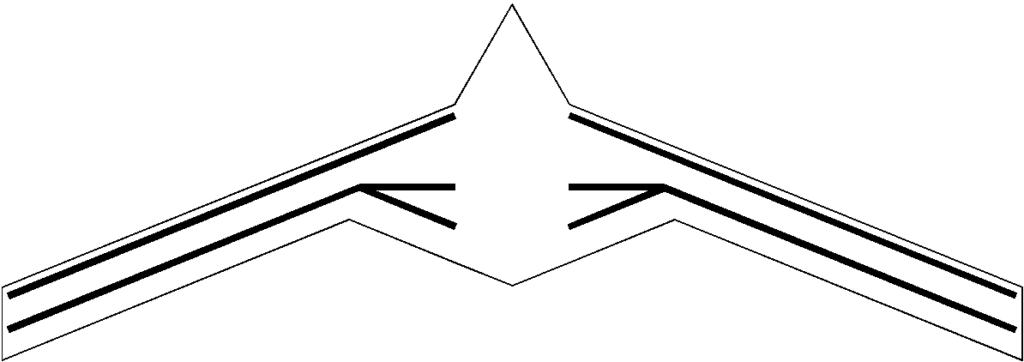
Engineers installed a two-line fiber system on both the top and bottom wing surfaces of the X-56A to simultaneously monitor the bending and cross-sectional rotations of each wing. The distributed strain data was acquired along the wingspan and used to derive the oscillation velocity of the wings which is indicative of flutter onset. As each fiber line is comprised of hundreds of sensing points, the FOS setup provided significantly more data and greater insight than traditional sensors.
Over the course of testing, the largest deflections occurred at the wing tips and the largest bending strains occur a few feet outboard the wing root. Historically, obtaining this level of insight into the overall strain distribution was only possible computationally through finite element models (FEM). Using FOS, finite element-like data is acquired experimentally on the actual structure. This allows critical areas to be confidently identified instead of just predicted, as well as serving as thorough FEM validation.
A histogram of bending strain and deflection data acquired through the leading-edge fibers over the duration of the FOS test flight. The x-axis corresponds to the sensor number corresponding to a discrete location along the leading-edge fiber on the. The color indicates frequency of that level in the data, where pink is the mean over time and blue is less frequent. (Image courtesy: Sensuron)
A repackaged version of Sensuron LLC’s RTS125+ real-time FOS interrogator flew onboard the X-56A to acquire the strain and deflection data. Considering the specific operating environment, the RTS125+ system’s size and weight was modified – while still offering a level of ruggedization – to ensure optimal performance throughout the mission.
A repackaged version of the RTS125+ FOS interrogator flew onboard X-56 to acquire the FOS data. The overall weight of the interrogator (orange chassis) was reduced from 13 pounds to 6.7 pounds. (Image courtesy: Sensuron)
Sensuron’s RTS125+ acquires continuous strain and temperature distributions on up to 320 feet of small, virtually weightless, and long-lasting fiber optic cable. Optimized for flight applications at AFRC, this technology provides test and instrumentation engineers a new level of insight into the strain distributions, fatigue life, and in-operation performance of aerospace structures.
In addition to real-time, in-flight structural health monitoring, there are a multitude of applications within the aerospace industry where FOS technology is being utilized, including the subcomponent level through full-scale structural testing, crack detection, composites embedment and cure monitoring, finite element validation, flight loads monitoring, and many more. For applications that necessitate the use of hundreds or even thousands of electric strain gauges, a significant reduction in installation time, weight, and complexity is realized by switching to distributed FOS technology.
For smaller scale applications, significantly more sensors can be installed in the same amount of time that it takes to install only a few traditional strain gauges. Regardless of the application, fiber optic sensing technology enables engineers to capture significantly more data than they can practically with traditional sensors.